There are two sides of energy metabolism. While the first covers energy production activities from foods; The second includes all activities that consume energy in the body. This article describes the production of power from food in the body.
One of the most important reasons we consume food is to provide energy to the body. The food eaten is primarily digested; Then, the resulting building blocks are given to the blood to reach all the body cells. These building blocks are used for all activities carried out in cells.
When the building blocks are used for energy production, the energy released is transferred to the produced adenosine triphosphate (ATP) molecules. ATP can be thought of as energy-carrying packets and ATP is used in all energy-requiring activities.
The body can provide energy from carbohydrates, proteins and fats. The primary source used to meet the energy need is glucose, a carbohydrate monomer. While approximately 80% glucose is obtained from the carbohydrates consumed, 20% is composed of other carbohydrate monomers (fructose, galactose, mannose).
Although fructose is also a carbohydrate monomer, it must first go through some liver processes to obtain energy from fructose. Therefore, even fructose is not as convenient as glucose to get energy. Today, public health concerns about the use of corn syrup stem from this situation.
The body turns glucose into polymers in glycogen and stores it as a ready-to-use energy source when needed. The amount of glycogen stored in this way in the body is about 300 grams and it takes half an hour to deplete it in case of intense exertion.
Amino acids, which are protein monomers, are mainly used as building materials in the body. They are not used as an energizer except for intense effort or malnutrition. To obtain energy from amino acids, first of all, the amino group (-NH2) in the amino acid must be separated from the chain.
This process occurs in the liver (deamination) and muscles (transamination). The remaining carbon chain can provide energy; however, the leaving amino group (urea) must be immediately removed from the body. Urea is diluted with large amounts of water and excreted in the urine. This causes a large amount of water loss from the body and the body’s need for water increases.
Fat monomers are used as building blocks in the body and another important function of fat is to be the body’s main energy store.
During intense exertion, cells primarily use glucose from the blood; then glycogen is broken down and the released glucose is used; When glycogen is depleted, the destruction of triglycerides is stored in the cell and adipose tissue begins. Energy production is provided by burning the released glycerol and fatty acids.
The body can produce energy from nutrients in two different ways: aerobic and anaerobic respiration.
1. Energy production; Aerobic Respiration
Aerobic respiration releases the energy production they contain by burning carbohydrates, fat and protein building blocks with oxygen. The reaction takes place in 3 stages and as a result water, carbon dioxide and ATP are formed. These stages are;
– Preparation of nutrients for the Krebs cycle,
– Krebs cycle,
– It is the electron transport system (ETS).
While ATP production in the first two stages is carried out by “substrate-level phosphorylation”, ATP production in the third stage, the electron transport system, is carried out by “oxidative phosphorylation”. The amount of ATP produced by oxidative phosphorylation is much greater.
In aerobic respiration, oxygen is used as a hydrogen scavenger in the electron transport system. It would be more beneficial to explain the stages of aerobic respiration under their headings.
1.1. Preparation of nutrients for the Krebs cycle
Essentially, the Krebs cycle begins with the Acetyl CoA molecule. Glucose, amino acids, glycerol and fatty acids are prepared in specific ways and included in certain parts of the Krebs cycle.
Once glucose is taken into the cell, it is broken down into pyruvate in the cytoplasm. This reaction is called “glycolysis”. The glycolysis reaction is given below;

As a result of glucose undergoing glycolysis, two pyruvates, net 2 ATP and 2 NADH+ are produced. ATPs produced as a result of this reaction are obtained by substrate-level phosphorylation. The NADH+ produced is converted to ATP in the electron transport system.
For glucose to be included in the Krebs cycle, it is not sufficient to break it down to pyruvate. After this stage, the two-produced pyruvate is transported from the cytoplasm and brought to the mitochondrial matrix, where is converted into 2-carbon Acetyl CoA by losing carbon dioxide by the enzyme “pyruvate dehydrogenase”.
The reaction in which pyruvate is converted to Acetyl CoA is given below;
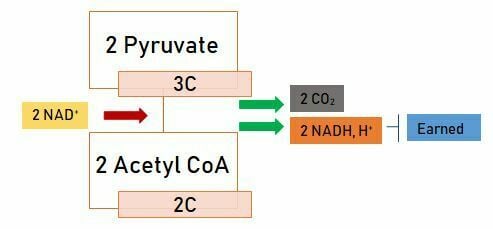
2 Acetyl CoA is obtained from 2 Pyruvates. 2 NADH+ is produced and transferred to the electron transport system during this reaction. Glucose is broken down to Acetyl CoA and is ready for the Krebs cycle.
When a fat molecule (triglyceride) is used to provide energy, first of all, the fat molecule is broken down in the adipose tissue. As a result of this breakdown, one glycerol and three fatty acid molecules are released and given to the blood to be delivered to the cells.
Depending on the triglyceride structure, the three released fatty acids may be the same fatty acid or maybe 2 or 3 different fatty acids. The situation that needs to be known here is that the chain length of the released fatty acid changes the amount of energy produced.
The amount of energy produced from butyric acid, which is 4 carbon fatty acid, and the amount of energy produced from stearic acid, which is 18 carbon fatty acid, are naturally not the same.
To return to the subject again, glycerol, which is released due to the destruction of a fat molecule, enters the glycolysis reaction just like glucose. As a result, pyruvate is produced. Pyruvate is also broken down into Acetyl CoA and in this way, glycerol is made ready for the Krebs cycle.
Fatty acids are subjected to “β-oxidation” in the mitochondria and as a result of this reaction, fatty acids are broken down and converted to Acetyl CoA. In this way, fatty acids are prepared for the Krebs cycle.
The important thing here is that oxygen is needed for the oxidation of fatty acids, as the name suggests. If there is not enough oxygen, fatty acids cannot be converted to Acetyl CoA.
As mentioned before, amino acids must first get rid of their amino groups to produce energy from amino acids. For this purpose, amino acids are subjected to deamination in the liver and transamination in the muscles.
The amino group is removed from the body; The remaining carbon chain is given to the blood and delivered to the cells. Since the molecular structures of amino acids are different from each other, there is no standard procedure for all of them in the cell.
Some amino acid chains are glycosylated; some are directly converted to Acetyl CoA; others enter the cycle from different points of the Krebs cycle.
1.2. Krebs Cycle (Tricarboxylic acid (TCA) cycle, Citric acid cycle)
In the process up to this stage, the building blocks of nutrients have been converted to Acetyl CoA. Other names for the Krebs cycle are the tricarboxylic acid (TCA) cycle and the citric acid cycle. The diagram of the Krebs cycle is given below;

Acetyl CoA combines with 4-carbon oxaloacetate through the enzyme “citrate synthase” to form citrate and this is how the Krebs cycle begins. As a result of the cycle, 1 ATP, 3 NADH+, and 1 FADH2 are produced from one Acetyl CoA molecule. The produced NADH+ and FADH2 molecules are sent to the electron transport system.
It has been previously stated that 2 Acetyl CoA molecules are formed from 1 glucose molecule. 2 Krebs cycles are performed for one glucose molecule. Therefore, the incoming and outgoing products in the diagram must be multiplied by 2.
1.3. Electron Transport System (ETS)
Elements of the electron transport system are located in the inner membrane of the mitochondria and energy production is carried out in the inner membrane folds known as “cristae”.
There are two main operations in ETS. The first is the separation of the hydrogen ions in the NADH+ and FADH2 molecules obtained in the process up to this stage and the combining of the separated hydrogen ions with oxygen.
The second is the conversion of the potential energy, which is formed by hydrogen ions and the density difference on both sides of the membrane, into chemical energy (chemiosmotic hypothesis).
NADH+ and FADH2 molecules are separated from the H+ ions they contain and the free NAD and FAD molecules are returned to the system for reuse. The oxygen taken from the lungs with aerobic respiration is responsible for keeping the H+ ions here, resulting in H2O; that is, water is formed. If there is not enough oxygen, the Electron Transport System will not work.
The chemiosmotic hypothesis explains energy production by the electron transport system. This hypothesis was not accepted when it was first put forward (1961); however, when supportive results were obtained in the studies carried out in the following years, it was adopted by the scientific world and won the Nobel Prize in Chemistry in 1978.
According to the chemiosmotic hypothesis, the H+ ions separated from the NADH+ and FADH2 molecules accumulate outside the inner membrane, resulting in a difference in ion density between the inside and the outside.
The resulting density difference means potential energy. This potential energy is converted into chemical energy as ATP by ATP synthase. Energy production in ETS is oxidative phosphorylation.
In this system, 2,5 moles of ATP from every 1 mole of NADH+; 1,5 moles of ATP can be produced from every 1 mole of FADH2.
A total of 10 NADH+ and 2 FADH2 are obtained during glycolysis from one glucose molecule, conversion from pyruvate to Acetyl CoA and the Krebs cycle. As a result of using these molecules in ETS, a total of 28 ATPs are produced.
2. Energy production; Anaerobic Respiration
Energy production by anaerobic respiration occurs in the muscles during intense physical activity. The energy efficiency is relatively low; however, it is essential to meet urgent energy needs.
Glucose is burned by anaerobic respiration and as a result, 2 moles of ATP and 2 moles of lactic acid are released. The reason why anaerobic respiration is called lactic acid fermentation is that lactic acid is released due to the reaction. Fatigue during physical activity is due to the accumulation of lactic acid.
Amount of Energy Production
As a result of aerobic respiration, a total of 32 moles of ATP is produced from 1 mole of glucose (180 grams). The table regarding the production is given below. Essentially, there is the potential to produce about 85 moles of ATP from 1 mole of glucose; however, the amount produced is 32 moles and the remaining energy is released as heat.
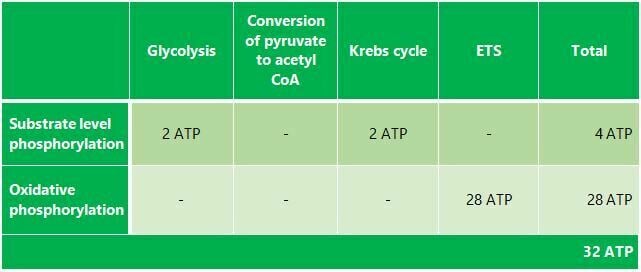
As a result of the combustion of 1 mole of glycerol, one of the building blocks of triglycerides, 19 moles of ATP are produced with oxygen respiration. As mentioned before, fatty acids release energy at varying levels in proportion to the number of carbons they contain.
As a result of burning 1 mole of stearic acid, 148 moles of ATP are produced. Depending on the nature of a triglyceride, 50 – 580 moles of ATP can be produced per mole of triglyceride.
If we look at the energy production from proteins, an average of 40 ATP can be produced due to burning amino acids.
Although the energy unit is ATP in terms of cell chemistry, different energy units express the energy amounts of foods in daily life. The first of these units is “calorie”. A calorie is the amount of energy required to raise the temperature of one gram of water from 15oC to 16oC and is denoted by “cal”.
Another energy unit used to indicate the energy value of foods is the “joule”. One joule is the amount of energy required to carry a kilogram of weight one meter with a force of one newton and is denoted by “j”. 1000 calories (cal) equals 1 kilocalorie (kcal). 1 kcal is 4.184 kJ. 1 mole of ATP provides 7,3 kcal of energy.
The calorie unit is actually universally misused. The amount we express as calories in daily life is actually kilocalories. For example, when it is said that “an apple contains 100 calories”, it actually means that it contains 100 kilocalories.
This error is almost the same as “I bought 2 grams of tomatoes from the grocery store”. This misuse resulted in a more wrong solution and the term calorie was called “small calorie”. This expression is similar to saying “small gram” to gram. On food labels, calorie is used as a unit of energy; but its symbol is “kcal”.
As a result, the energy amount of foods is expressed by “how many calories a gram contains”. Experimentally, 1 gram of carbohydrate gives 4,1 kcal, 1 gram of protein provides 5,6 kcal and 1 gram of fat gives 9,3 kcal of energy.
However, efficiency in the experimental environment cannot be achieved in metabolism. In metabolism, 1 gram of carbohydrate gives 4 kcal, 1 gram of protein 4 kcal and 1 gram of fat provides 9 kcal of energy. If the amount of nutrients contained in a food is known, the total energy that that food will provide can be easily calculated based on these data.
For example, let’s say that 100 grams of a food contain 20 grams of carbohydrates, 12 grams of fat and 17 grams of protein. The energy that 100 grams of this food will give;
For carbohydrates; 20 x 4 = 80 kcal
For oil; 12 x 9 = 108 kcal
For protein; 17 x 4 = 68 kcal
TOTAL; = 256 kcal
One hundred grams of the food in the example gives 256 kcal of energy. Components such as water, vitamins, or minerals are not considered in the energy calculation. As a matter of fact, nothing gives energy except carbohydrates, fats and proteins.
Regarding carbohydrates, it should be noted that indigestible dietary fibers do not provide energy either. Alcohol is out of scope.
Here’s an article that might interest you;
Energy Metabolism – II: Energy Expended by the Body
Be First to Comment